Academic Experiences
Research Assistant in “Water, Environment and Sustainable Development”, “Hydrometallurgy and Flotation”, “Polymer Science and Technology” and “Computational Chemistry” laboratories.
Mentoring a Master’s Dissertation: High-purity Barium Sulfate Synthesis using Acid Leaching.
Abstract: Barium sulfate finds widespread application across various industries, each with distinct purity requirements. Despite Iran’s abundant natural barite deposits, the country faces a scarcity of high-purity barium sulfate, necessitating imports for numerous sectors. This study addresses these limitations by exploring two methods for producing high-purity barium sulfate: acid leaching and synthetic production. In the initial phase, we delved into the acid leaching process’s effectiveness in purging natural barite of impurities. We scrutinized key parameters including acid concentration, solid percentage, solution temperature, leaching duration, and stirring speed. The starting sample density stood at 4.328 g/cm3. Primary cleansing involved using a 30% volume of hydrochloric acid, followed by fuming sulfuric acid with concentrations ranging from 55% to 95% by volume (a blend of water and acid) for secondary purification. Hydrochloric acid proved effective in eliminating barite impurities, as barite remains insoluble in hydrochloric acid. After this procedure, the density increased to 4.345 g/cm3. Nonetheless, the results indicated that washing barite with fuming sulfuric acid was ineffective at removing impurities, leading to a density decrease to 4.313 g/cm3. Subsequently, sulfuric and hydrochloric acids were employed for leaching, with hydrochloric acid demonstrating superior efficiency in impurity removal. In the second phase, we pursued the synthesis of barium sulfate by combining barium chloride with sodium sulfate, ammonium sulfate, and sulfuric acid. To optimize conditions while minimizing experimentation, we adopted a Design of Experiments approach with DX7 software. We identified input variables, including barium chloride concentration (ranging from 0.35 to 1.5 M), sulfate concentration (ranging from 0.35 to 1.5 M), yield rate (2.5 to 20 cc/min), and temperature (20°C to 80°C). The output variables encompassed the mass and density of barium sulfate. Our findings indicated that sulfate and barium chloride concentrations exerted the most significant influence on the produced substance’s mass. Notably, the mass of barium sulfate exhibited relative independence from yield rate and temperature variations due to the rapid reaction kinetics. In summary, elevating barium chloride concentration, reducing sulfate ion concentration, and lowering the temperature and yield rate collectively contributed to enhanced barium sulfate density.
Effect of Carbon Nanofiber on the Mechanical Properties of Epoxy Nanocomposites
Abstract: Various nanofillers can significantly enhance the mechanical properties of composite materials. To achieve this, fibers must exhibit high modulus and strength (comparable to ceramics and metals) while maintaining low density. Composites typically consist of two main components: the matrix (or base) and the reinforcement. In this study, the effect of incorporating different mass percentages of ultralight hollow carbon nanofibers on the mechanical properties of two types of epoxy resins was investigated. The epoxy resins used were LR-630 with hardener LH-630 and ML-506 with hardener HA-11. Eight dumbbell-shaped samples were fabricated for tensile testing to measure properties such as elastic modulus, yield strength, tensile strength, and ductility. The goal was to improve the mechanical performance and reduce the weight of the composite material compared to pure epoxy resins. This research was conducted in the Polymer Science and Technology Research Laboratory at the University of Kashan between March and September 2020.
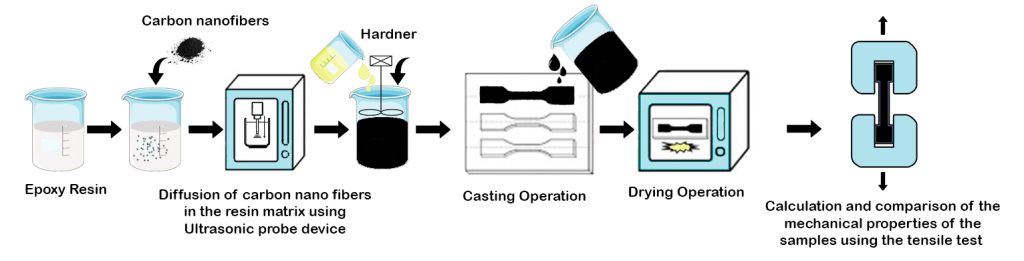
Student Intern and R&D Assistant at Patchimi Co., Kashan, Iran (PVA & PVOH prod.).
I worked at, Patchimi Co., a manufacturer of polyvinyl acetate resins used in the textile and carpet industry. In my Internship, I researched the production of polyvinyl alcohol, which isn’t produced in Iran, and this proved to be an incredible practical learning experience.
Computer Skills
Laboratory Experiences
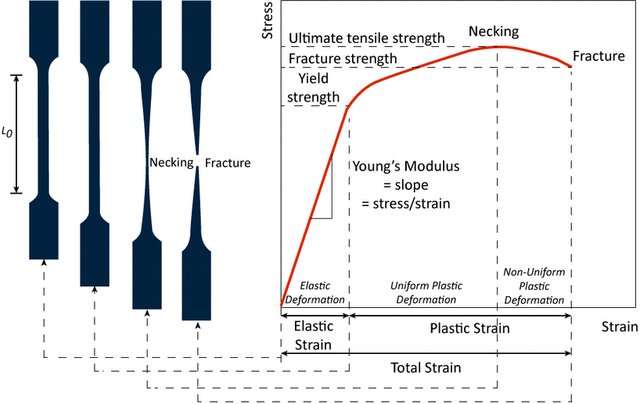
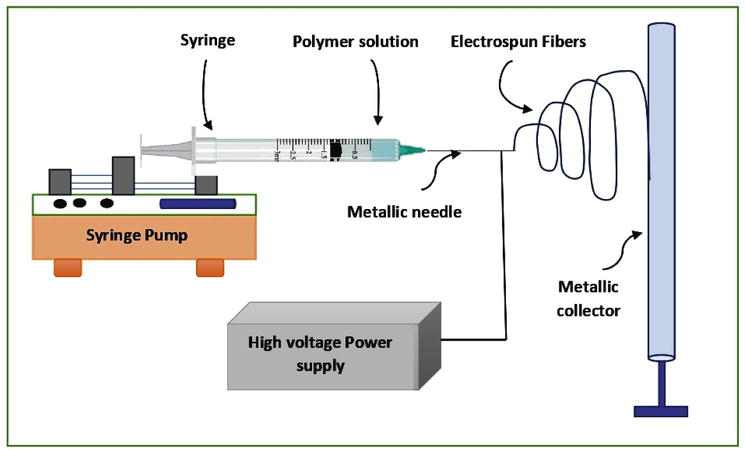
Test Scores
- IELTS academic test score: 7.5 (Listening: 8 – Reading: 8 – Writing: 6.5 – Speaking: 6.5)
- GRE test score: Verbal Reasoning: 140 – Quantitative Reasoning: 160 – Analytical Writing: 3
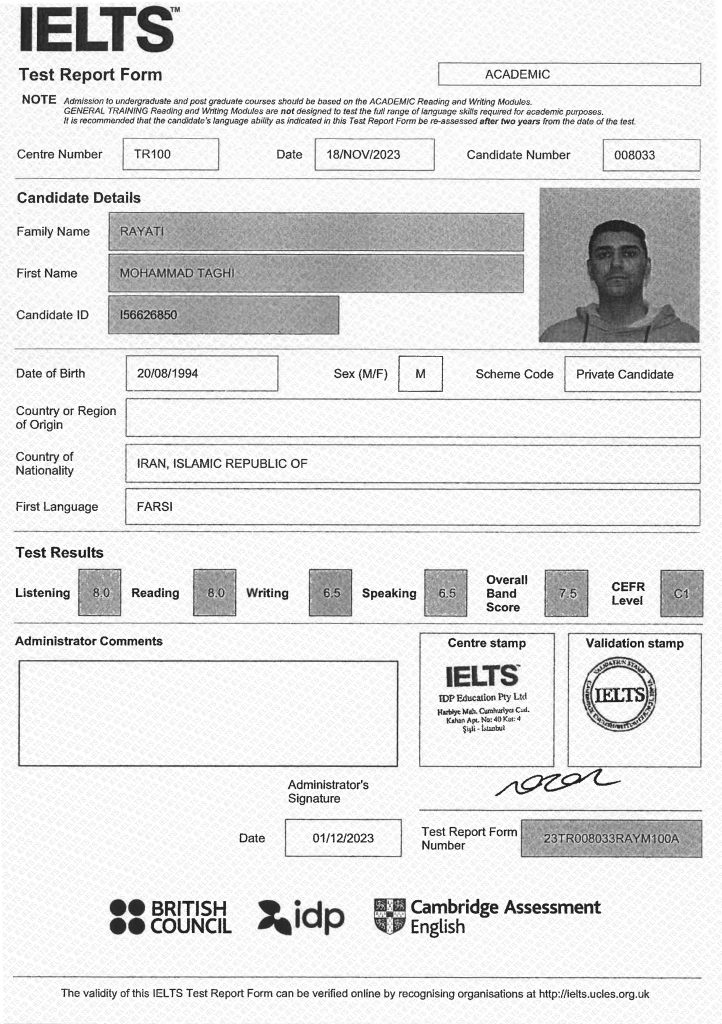
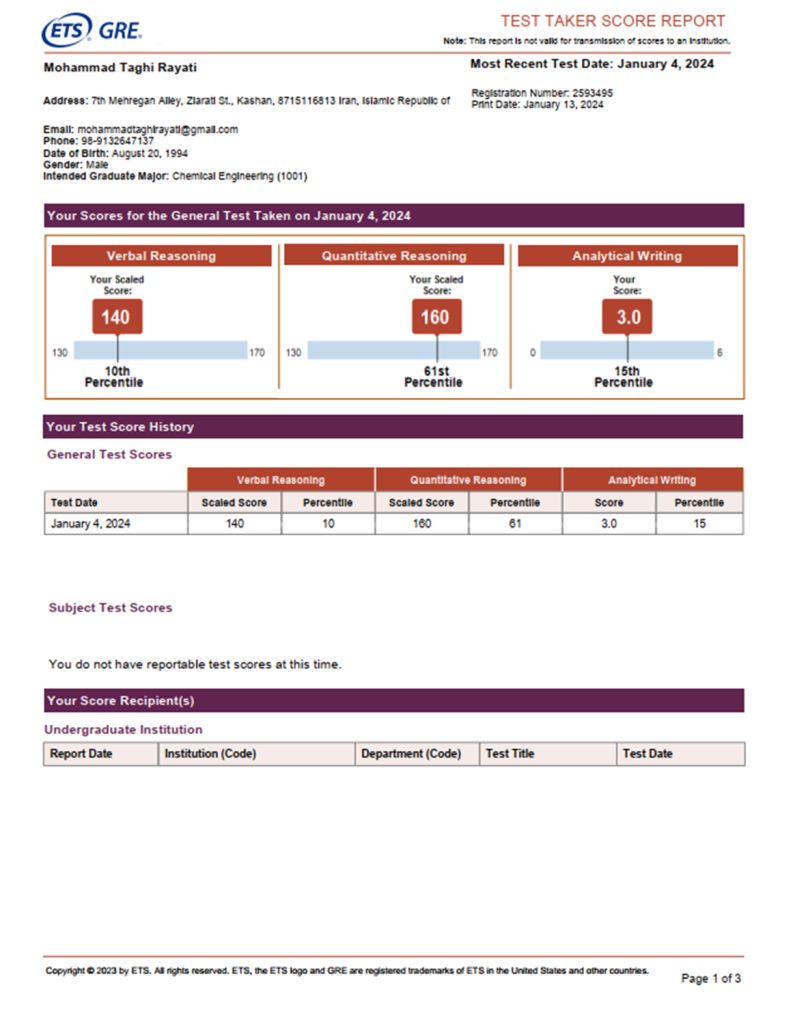
Molecular Simulation Projects
Determining the location of SO2 on the Ni(111) surface
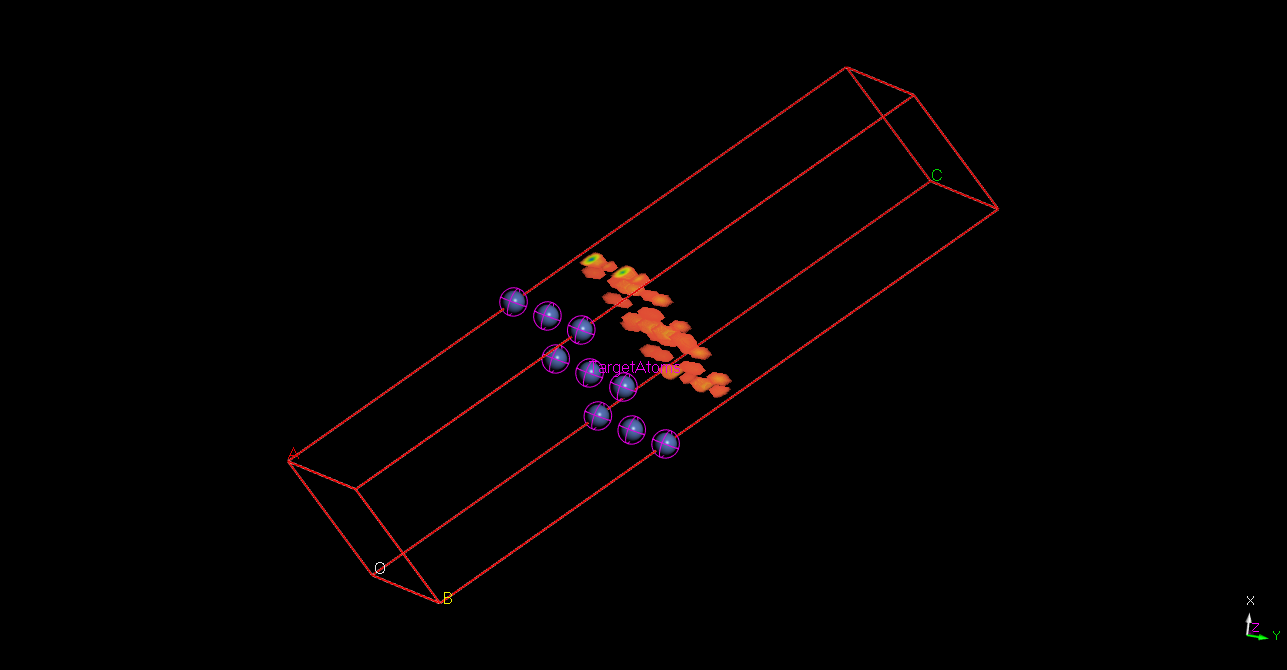
Modeling inhibitor (2-phenylglycine) adsorption onto a Pigment Red (diphenyl derivative of 1,4-diketopyrrolo(3,4-c)pyrrole, DPP) crystal face
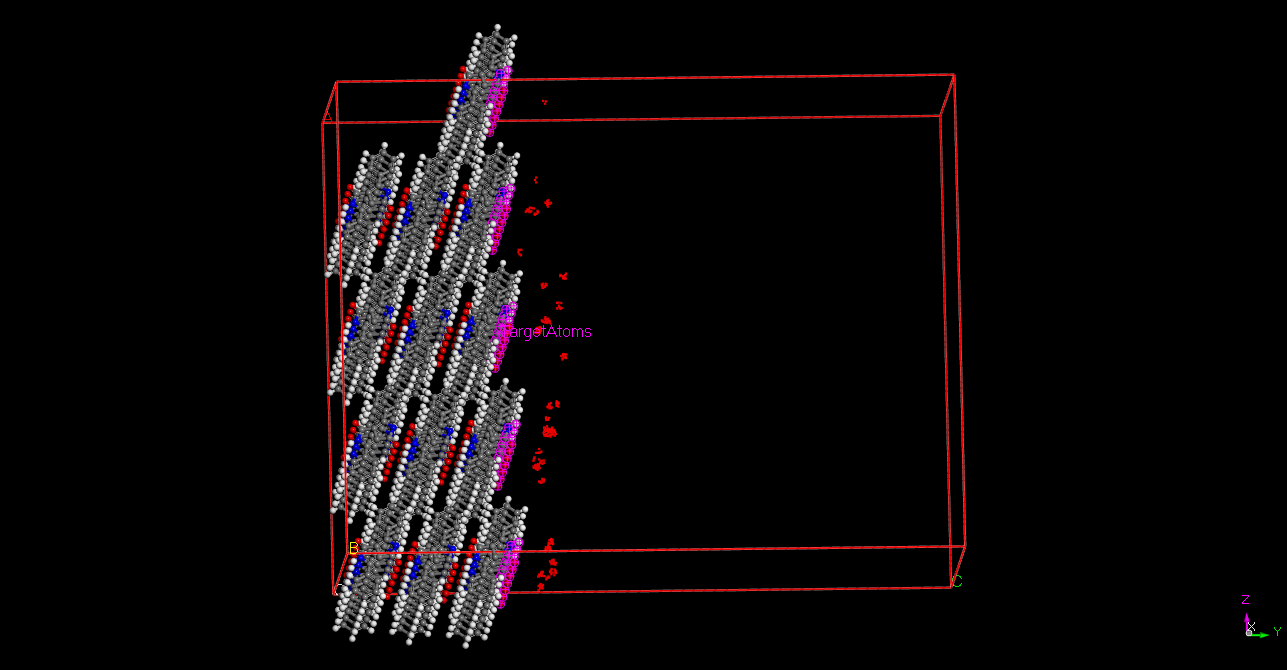
Packing molecules into existing structures

Geometry optimization of a carbon nanotube
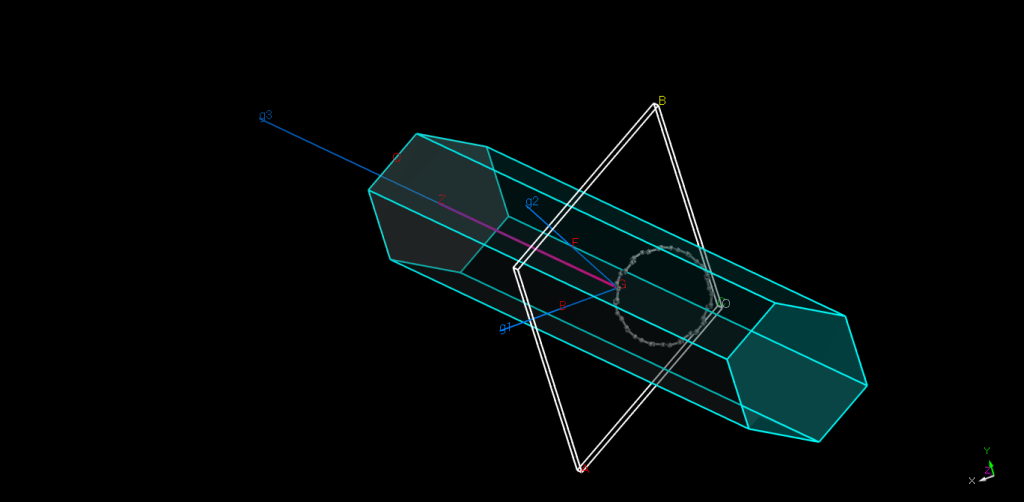
Creating parameters for DFTB+
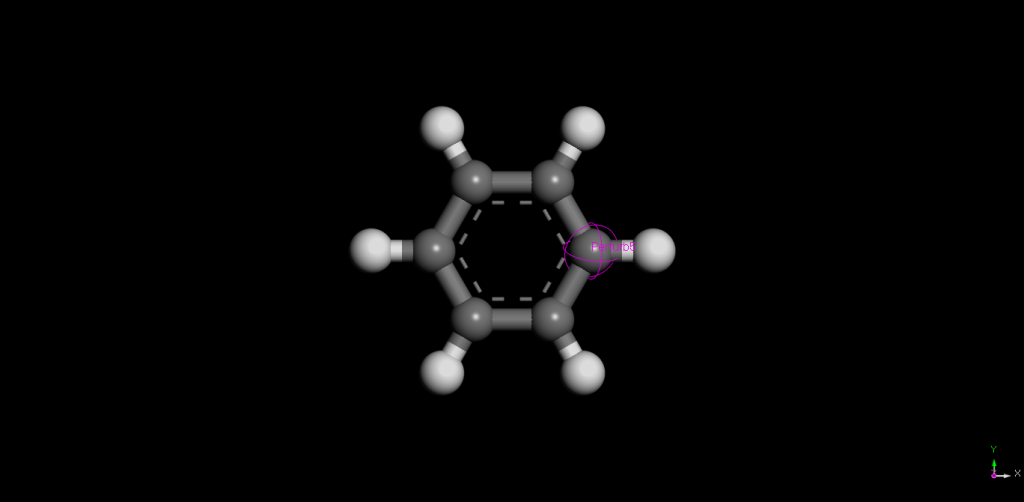
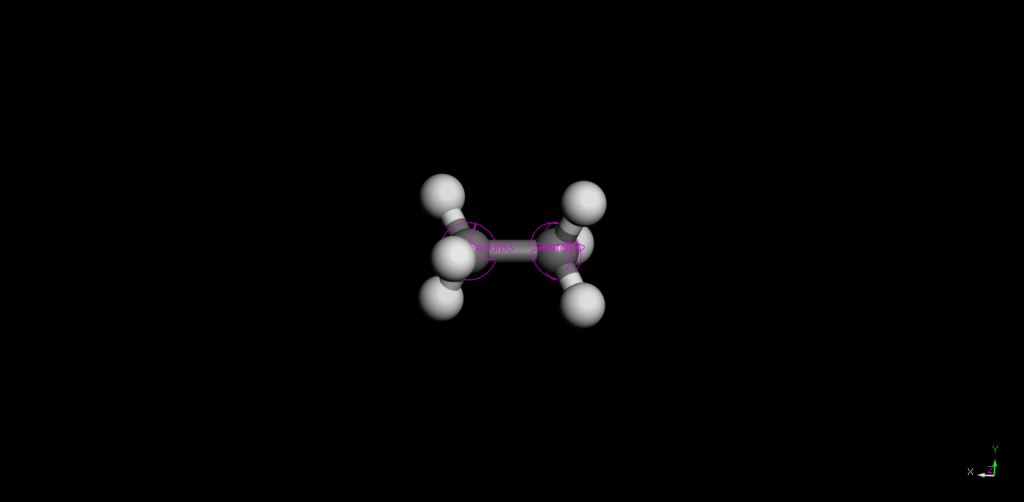
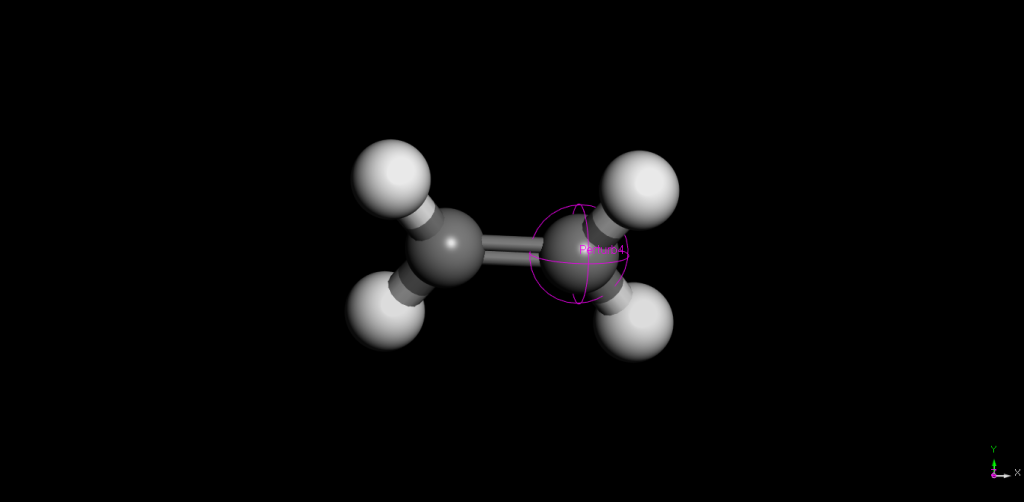
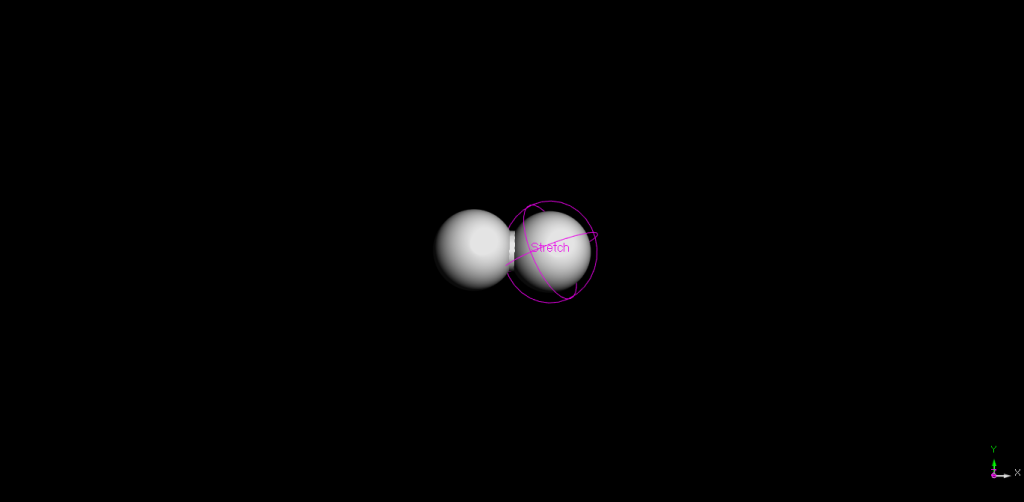
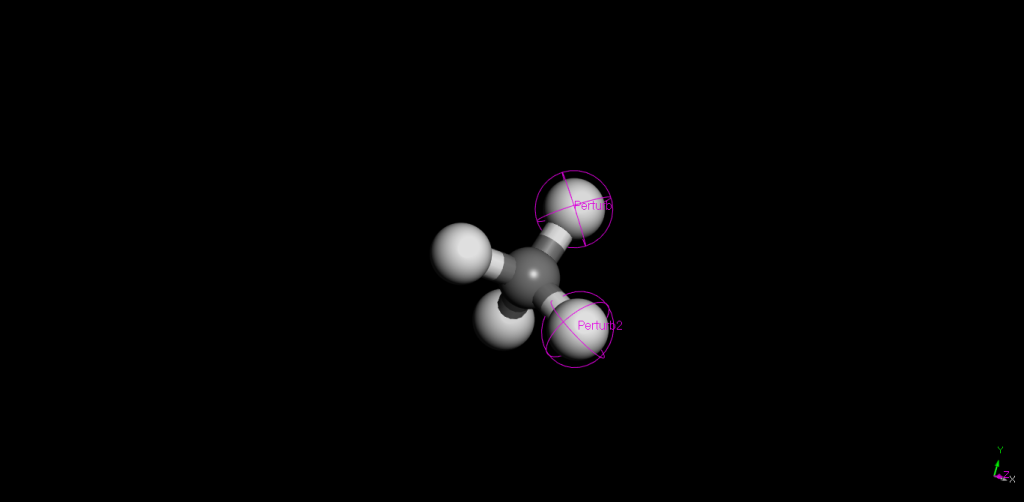
Simulating electron transport with DFTB+
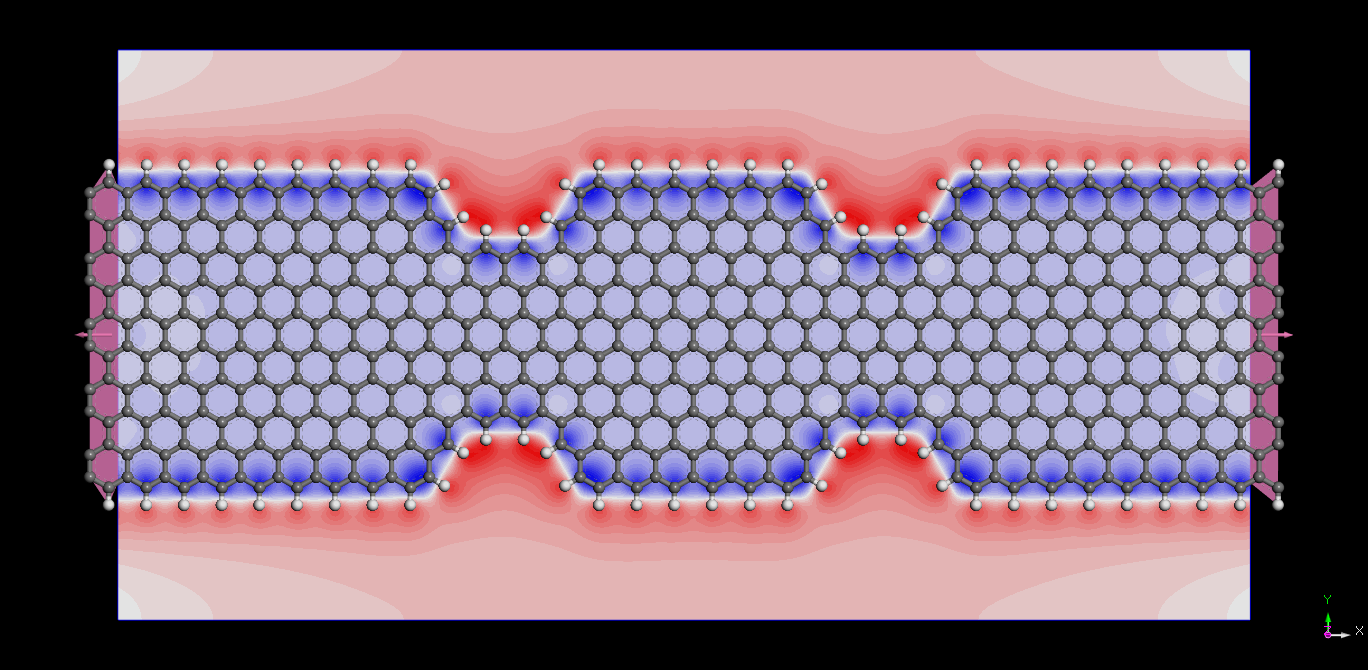
Computing band structure and density of states with DMol3
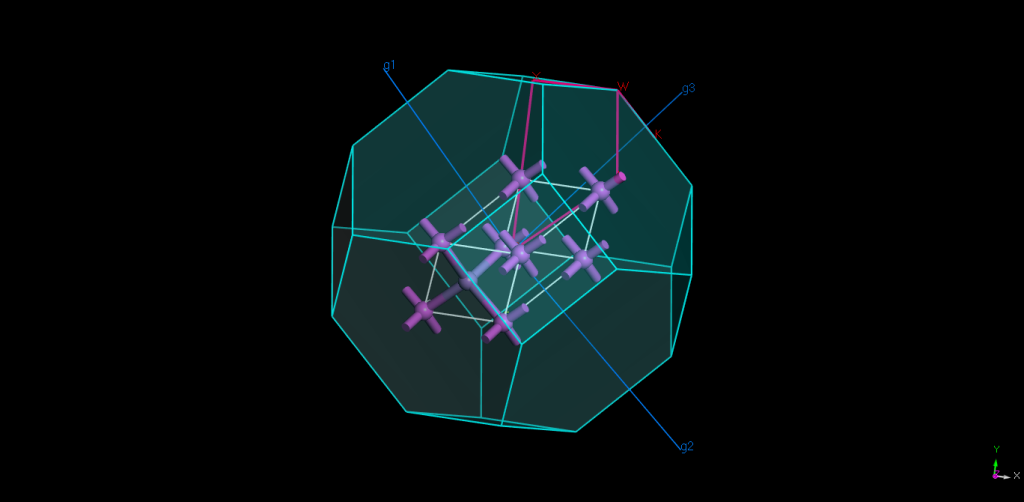
Geometry optimization of urea with and without symmetry constraints
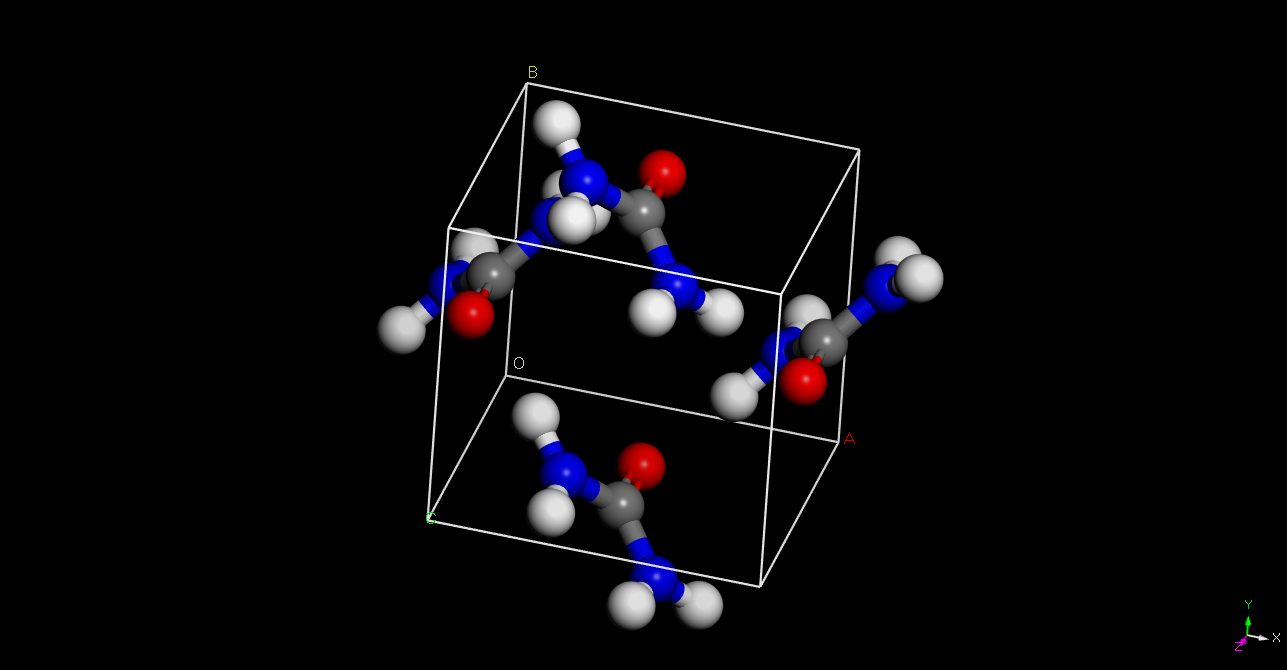
Hydrogen physisorption on a tungsten surface
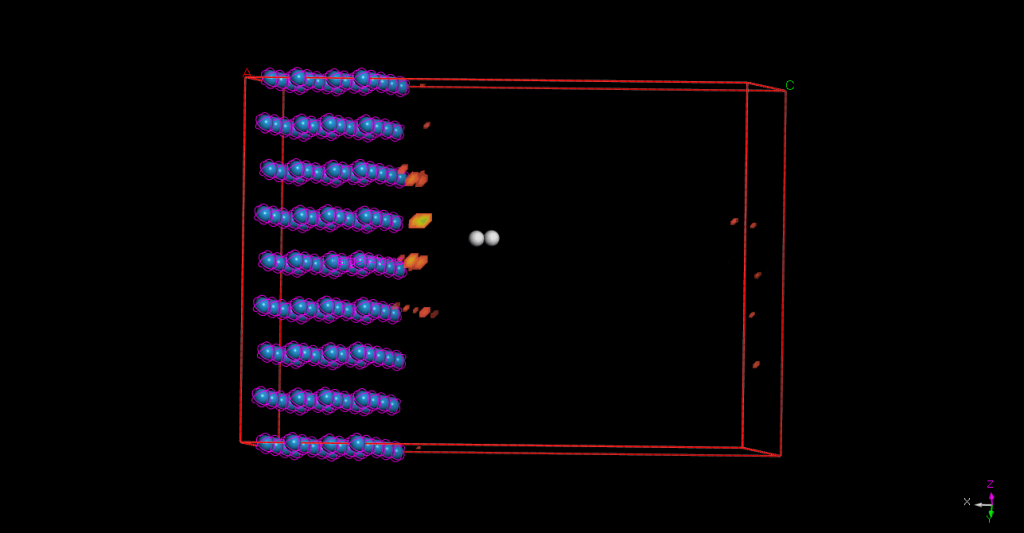
Calculating the diffusivity of a gas in a polymer
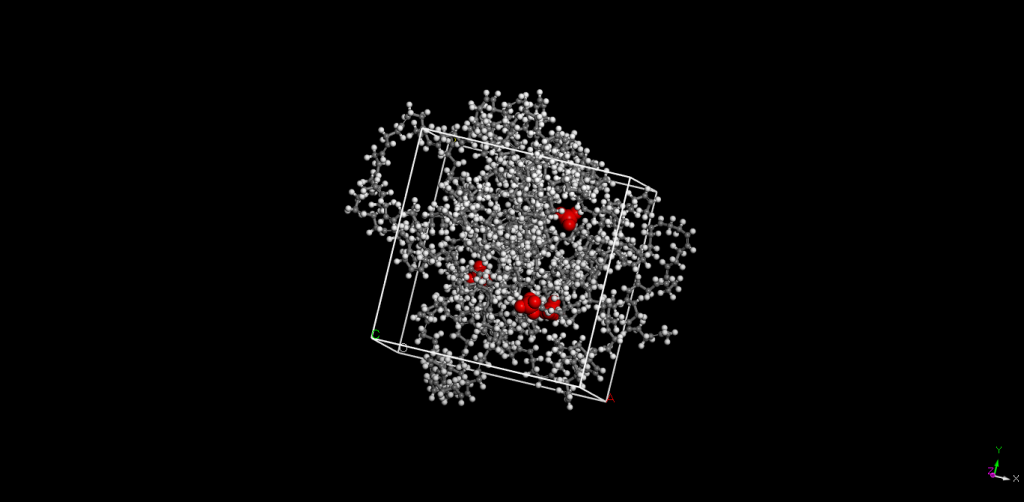
Interactions of Poly(p-nitrostyrene) with Al2O3 0 0 -1 plane surface
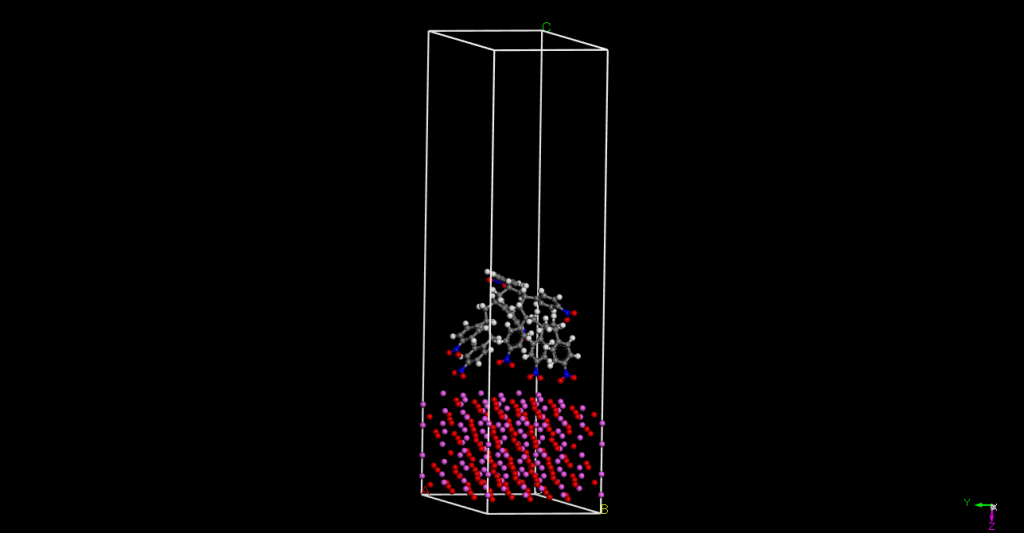
Calculating the miscibility of Polypropylene and Polystyrene

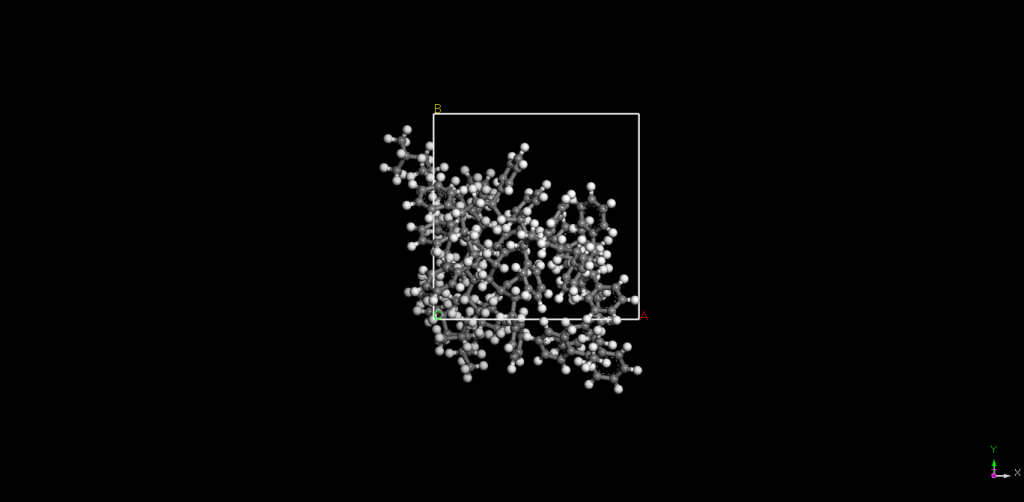
Running a confined shear simulation on Tridecane between two layers of Iron
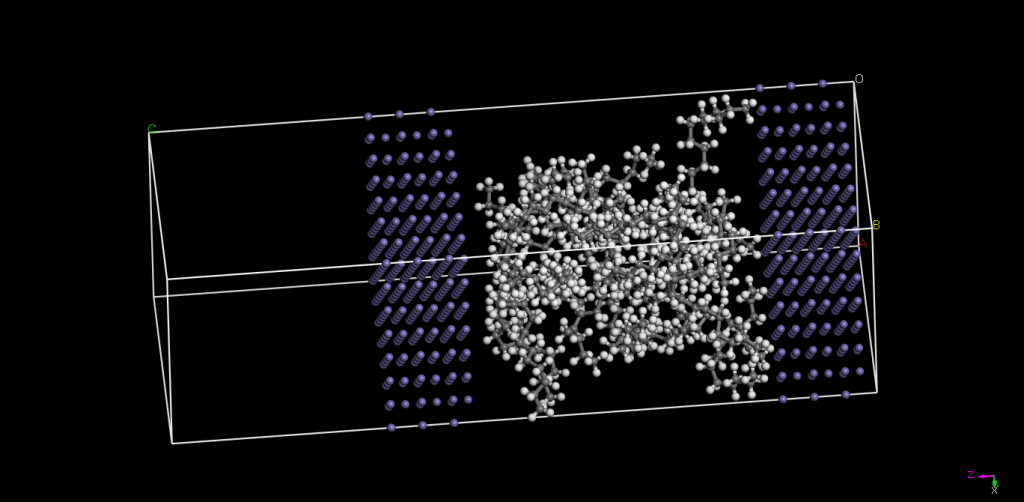
Calculating the solvation free energy of propionic acid in n-octanol
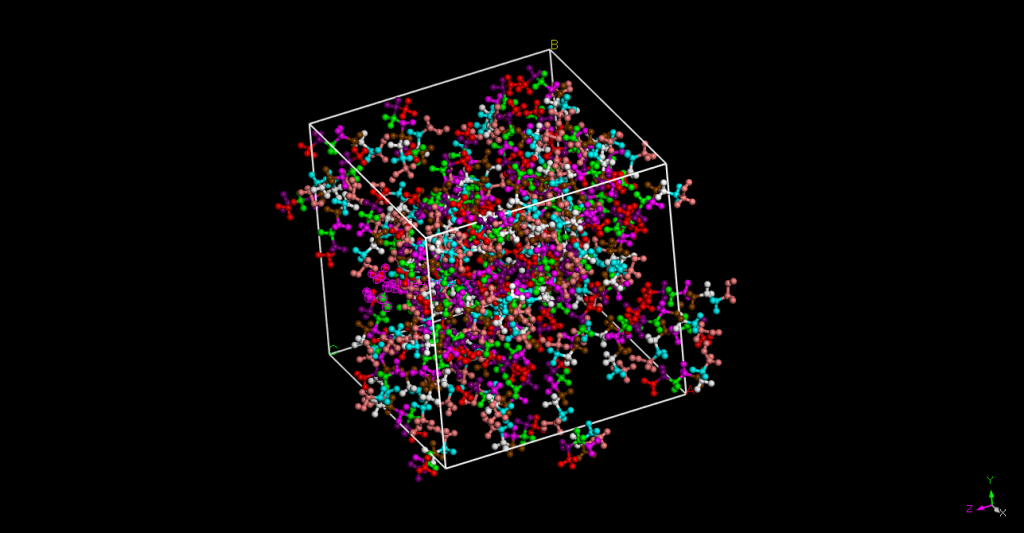
Course Certificates
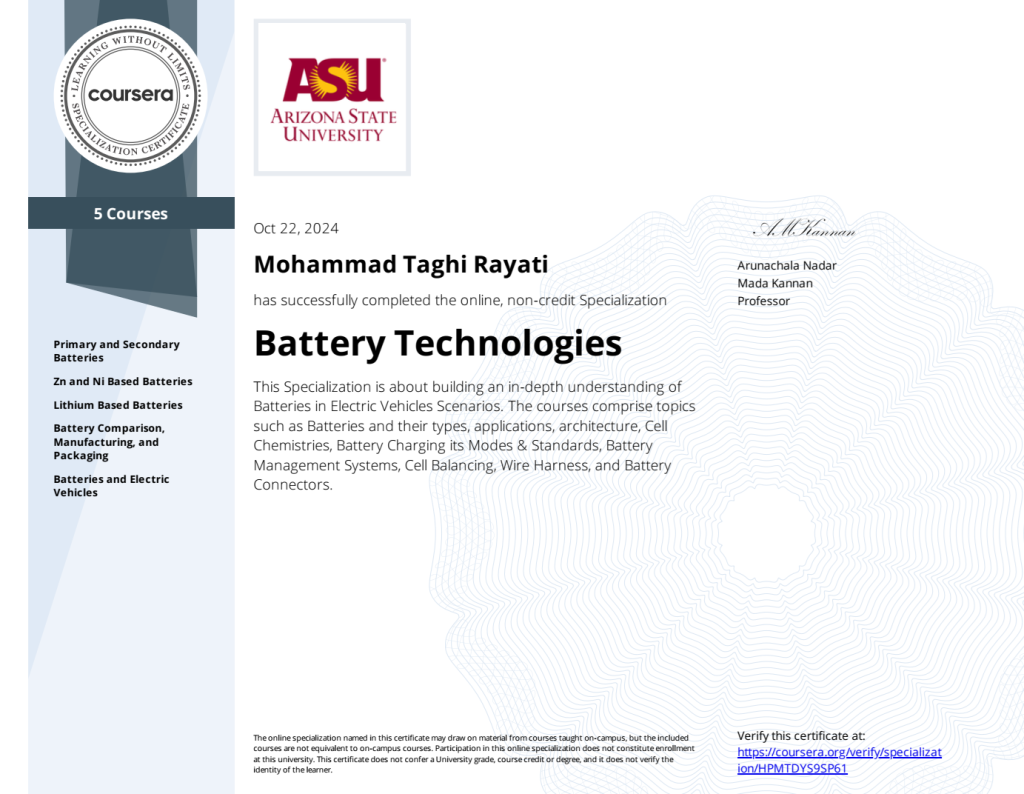
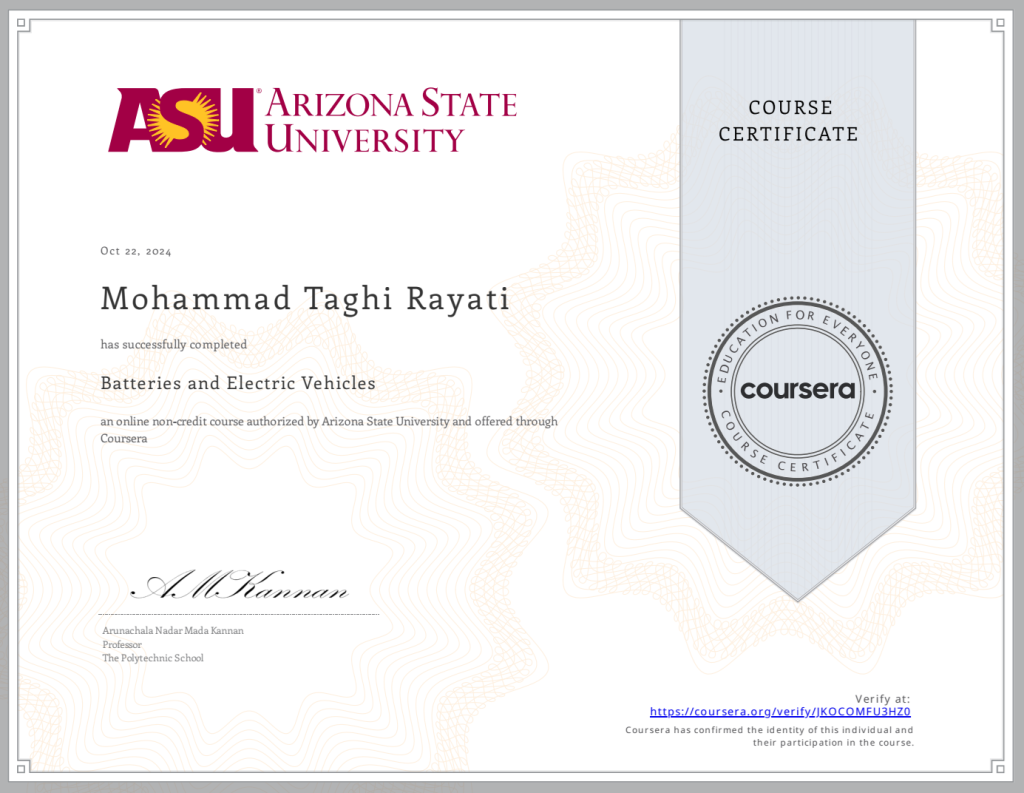
In the “Batteries and Electric Vehicles” course, I explored the critical role batteries play in electric vehicle (EV) technology, learning about different EV modes, including hybrid and battery electric vehicles. The course covered battery capacity, range, and performance, emphasizing the importance of temperature conditions, sensors, and monitoring systems to maintain reliability. I also studied EV range anxiety and strategies to mitigate it, including charging infrastructure and influencing factors. Furthermore, the course delved into lithium-ion and solid-state batteries, their development, density, and future potential. Recycling and second-life battery use were also key topics, alongside the challenges and benefits of recycling EV batteries.
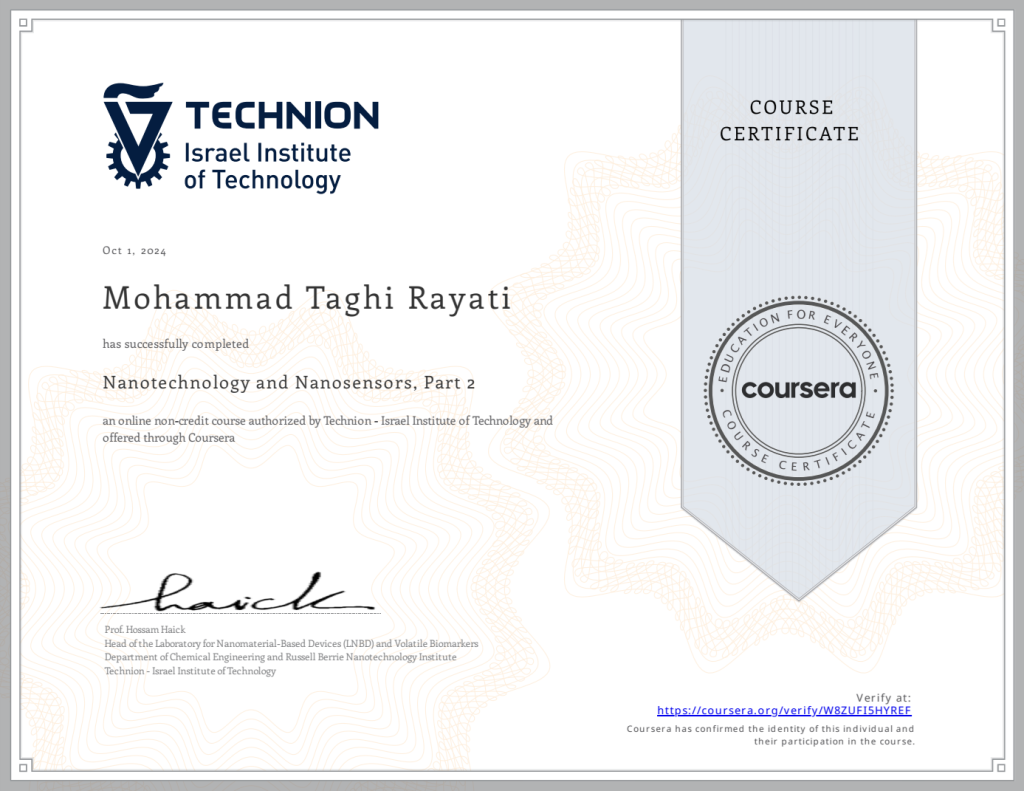
In the course ‘Nanotechnology and Nanosensors, Part 2,’ I explored various nanostructures and their sensing applications. I studied nanowires, including their properties, fabrication techniques, and usage in gas sensing and carbon nanotubes, examining their structure, synthesis, and applications in gas sensors. Metal oxide nanostructures were introduced, focusing on their classification, sensing mechanisms, and advancements in sensitivity. Polymeric nanostructures were also covered, emphasizing their synthesis and utilization in sensor development. Additionally, the course delved into the future of electronic skin, discussing designs incorporating nanowires, gold nanoparticles, carbon nanotubes, and field-effect transistors.
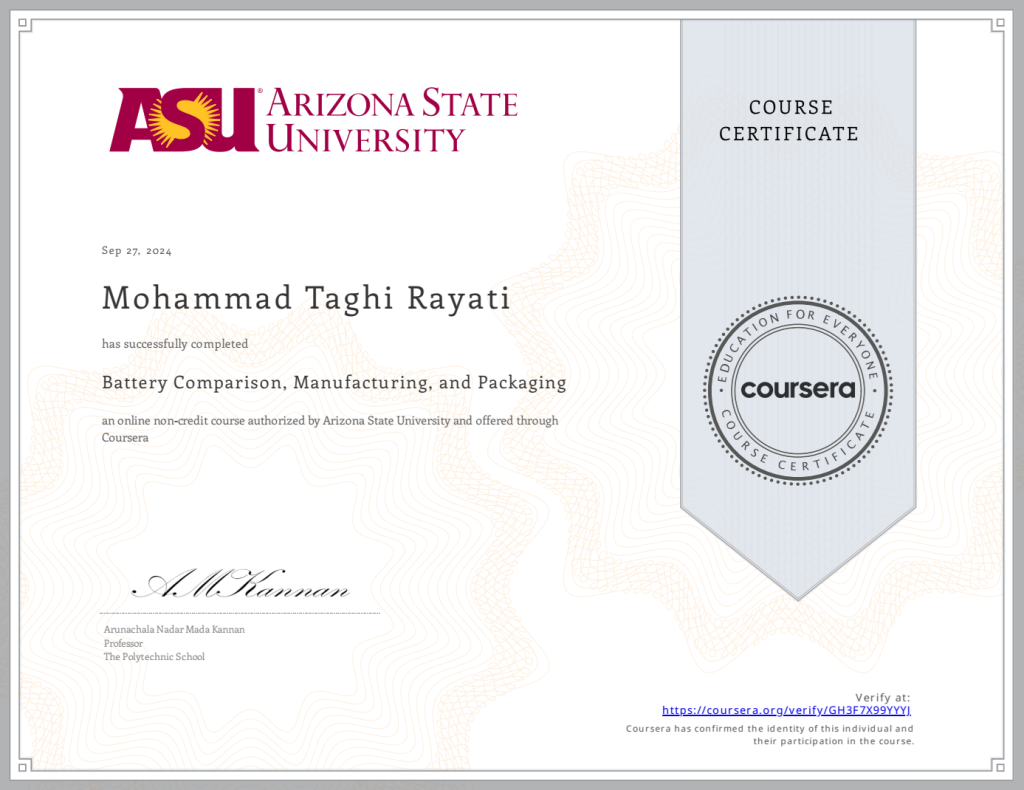
In the ‘Battery Comparison, Manufacturing, and Packaging’ course, I explored various battery technologies, including lead-acid, Ni-MH, and lithium-ion batteries (Li-ion). The course compared key battery types such as NMC (Nickel Manganese Cobalt) and LFP (Lithium Iron Phosphate), highlighting the wide-scale adoption of LFP due to its safety and longer cycle life. I also learned about the emerging benefits of solid-state batteries, which offer improved energy density and safety. Through a comparison of NMC, SLA (Sealed Lead-Acid), and LFP technologies, I gained a deeper understanding of the pros and cons of different battery chemistries and their suitability for various applications, including electric vehicles (EVs).
The course also delved into the intricate manufacturing process of EV batteries, focusing on components like Li-ion battery cells, modules, and packs. I learned about critical manufacturing stages, such as mixing, coating, drying, calendaring, slitting, and cell assembly. The course covered the importance of battery pack design, module configuration, and the role of Battery Management Systems (BMS) in ensuring safe and efficient operation. Additionally, I explored the importance of thermal management in EV battery systems and how the demand for EV batteries drives innovations in packaging and circular economy practices, contributing to sustainability in the industry.
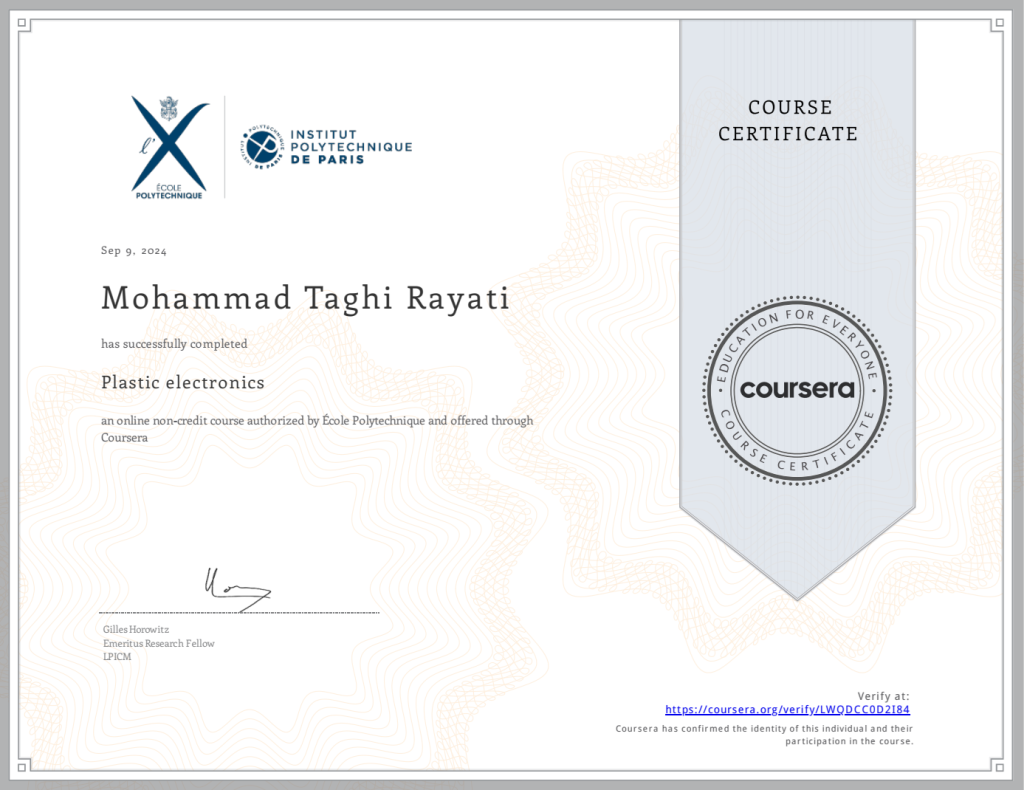
In the “Plastic Electronics” course, I explored the emerging field of organic-based electronic devices, focusing on the unique properties and behaviors of organic semiconductors. I learned about the fundamentals of charge transport in these materials, as well as how polarization and disorder can lead to the localization of charges. The course covered the optical properties of organic semiconductors, highlighting the role of excitons (bound electron-hole pairs) and their importance in devices like organic light-emitting diodes (OLEDs). I also studied energy levels, charge carrier injection mechanisms, and how to interpret current-voltage curves. Additionally, the course introduced key applications of plastic electronics, such as organic solar cells and organic field-effect transistors (OFETs), which offer flexible, lightweight alternatives to traditional silicon-based devices.
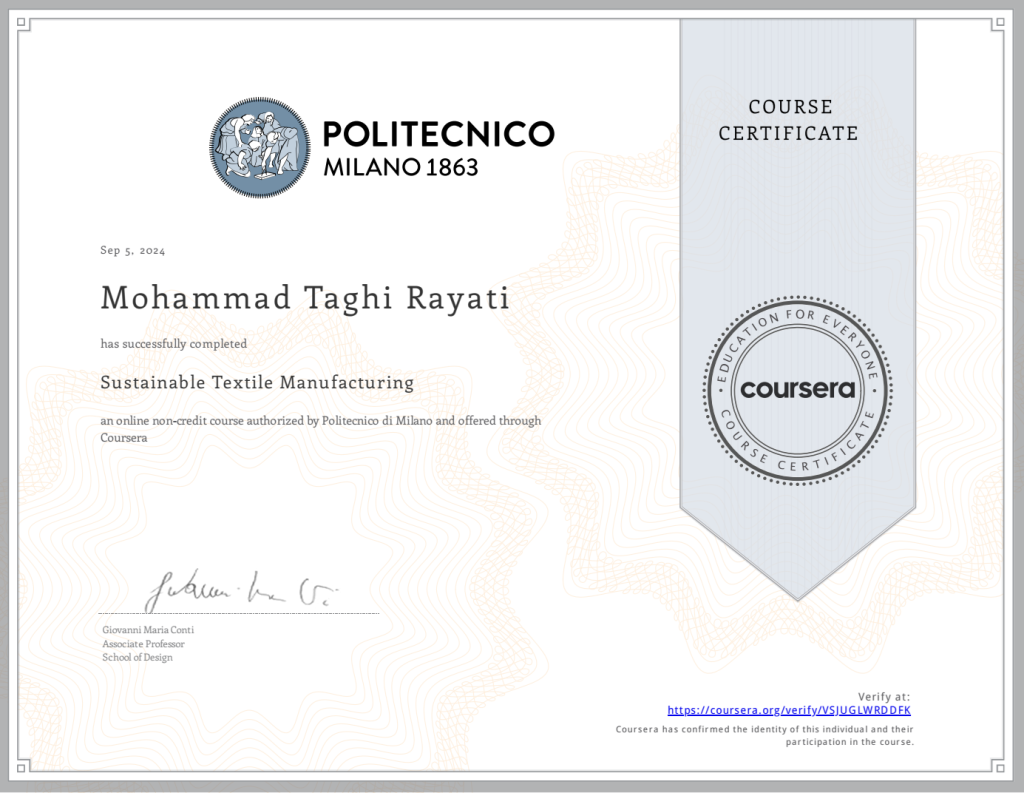
In the “Sustainable Textile Manufacturing” course, I explored the process of textile production with a focus on sustainability. I learned about traditional and new sustainable raw materials, and examined the processes of spinning and weaving, supported by case studies like Zegna Baruffa Lane Borgosesia for spinning and Vitale Barberis Canonico for weaving. The course also covered the design phase, prototyping, and production, including a design case study. Additionally, I studied how innovative technologies are integrated into textile manufacturing, with a specific case study on Shima Seiki’s advanced techniques.
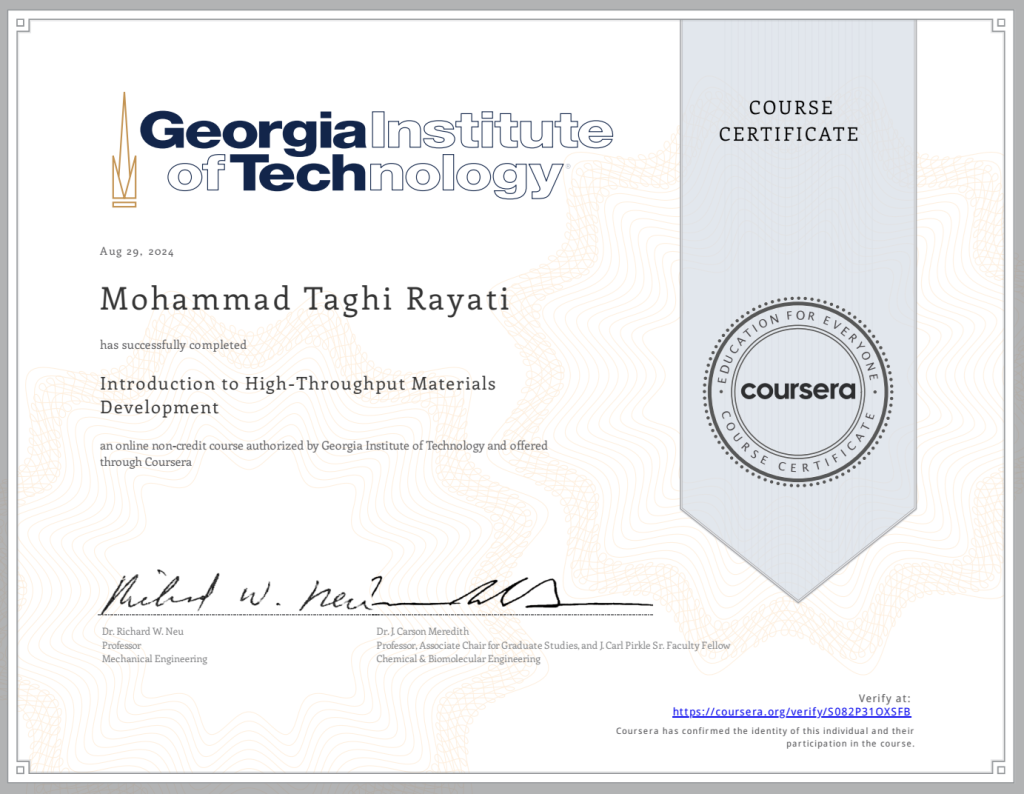
In the “Introduction to High-Throughput Materials Development” course, I explored the Materials Genome Initiative (MGI) approach and how high-throughput materials development (HTMD) fits into modern materials design. I learned about the complexities of designing materials and the history that led to the development of HTMD techniques. The course covered various high-throughput strategies, including computational screening, library preparation, and model-based experimental design. Key topics included the synthesis and processing of polymers, additive manufacturing, and metal alloy libraries, with a focus on creating gradient microstructures through techniques like vapor deposition, non-uniform heating and cooling, and rapid alloy prototyping.
I also studied high-throughput methods for characterizing material composition, structure, and properties. This included examining the optical, electrical, thermal, and mechanical properties of materials, such as strength, fracture toughness, and corrosion resistance. Advanced testing methods like instrumented indentation, abrasion, and scratch tests were introduced for polymers, coatings, and metals. The course applied these concepts to real-world applications, including proton exchange membranes for fuel cells, structural alloys for energy and transport, and property-structure-process linkages in dual-phase steel, emphasizing high-throughput prototyping and testing to accelerate materials discovery and development.
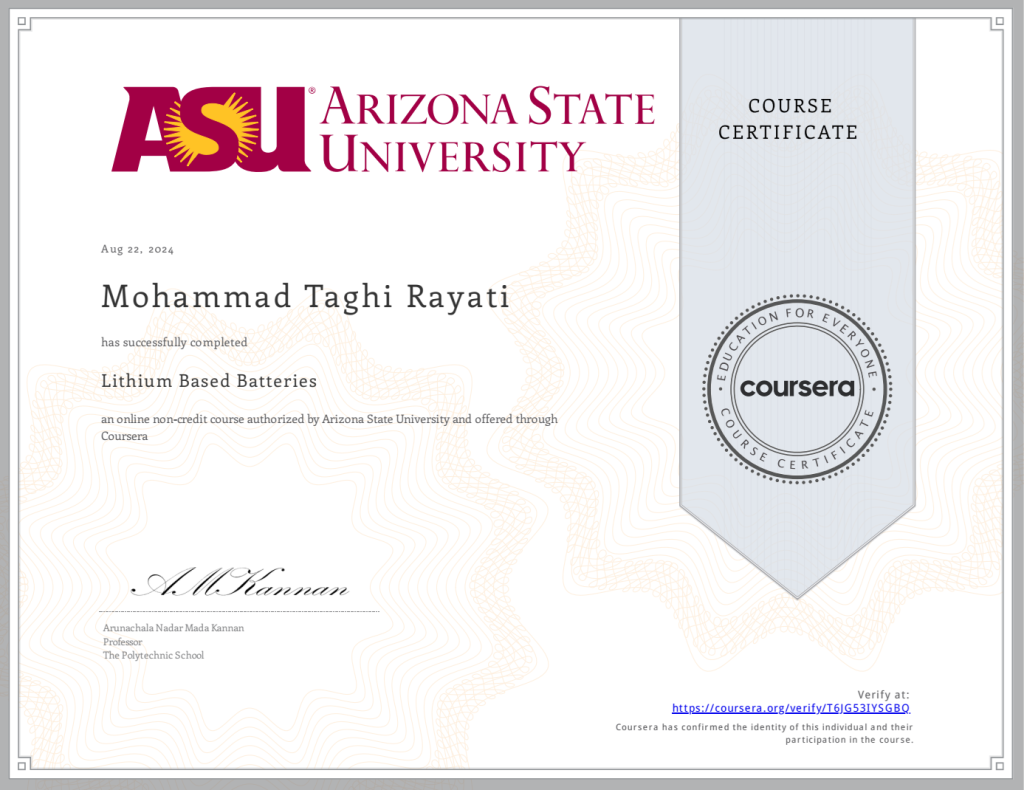
In the “Introduction to Lithium Based Batteries” course, I learned about the fundamentals of lithium battery technology, including the cell potential and the various applications of these batteries. The course provided an in-depth classification of lithium batteries, from lithium primary cells to advanced lithium-ion configurations. I studied specific battery types such as Li-FeS2, Li-MnO2, and Li-I2, focusing on their construction, performance, and key differences. Additionally, I explored the history and advantages of lithium-ion batteries, including their high energy density and efficiency, while also examining the materials used for anodes, cathodes, and electrolytes, and how these contribute to battery operation.
Further into the course, I delved into the challenges and innovations surrounding solid-state batteries, including the timeline of ionic conductors and development trends in battery technology. I explored the different types of solid-state batteries, such as those using solid polymer electrolytes and garnet-type oxides, along with the synthesis processes involved. The course highlighted ongoing technical challenges, particularly in battery companies, and looked at future applications and trends in the industry, emphasizing the importance of overcoming barriers in solid-state and lithium-ion battery technologies.
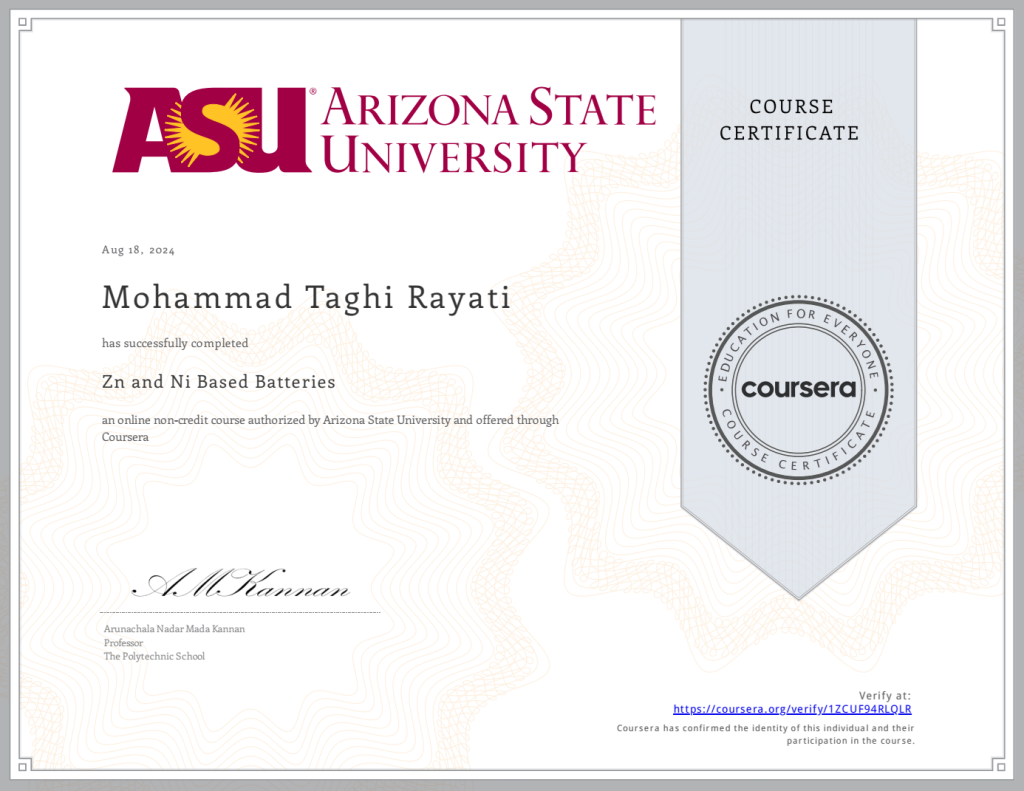
In the “Zn and Ni Based Batteries” course, I explored a variety of battery types, focusing on zinc and nickel-based systems. I learned about the materials used in battery cells, including the different anode and cathode materials, cell configurations, and their impact on capacity and energy storage. The course covered specific systems like the alkaline MnO₂ configuration and compared its performance with rechargeable Zn-MnO₂ and zinc-air batteries. Zinc-air batteries, in particular, were examined for their advantages, such as high energy density and applications in hearing aids and electric vehicles. I also learned about the growing market for zinc-based batteries, including rechargeable zinc-air and aqueous zinc-ion batteries, which are seen as more sustainable alternatives.
Additionally, the course introduced nickel-cadmium (Ni-Cd) and nickel-metal hydride (Ni-MH) batteries. I studied the materials, characteristics, and construction profiles of Ni-Cd batteries, noting their reliability and long life but also their environmental limitations due to cadmium toxicity. Ni-MH batteries were explored as a more environmentally friendly option, offering higher energy density but with some drawbacks like shorter lifespan and higher self-discharge. The course concluded with an overview of lead-acid batteries, including their electrodes, reactions, and the factors that affect their lifecycle. I compared these battery types, learning about their advantages, limitations, and applications in industries such as automotive and energy storage.
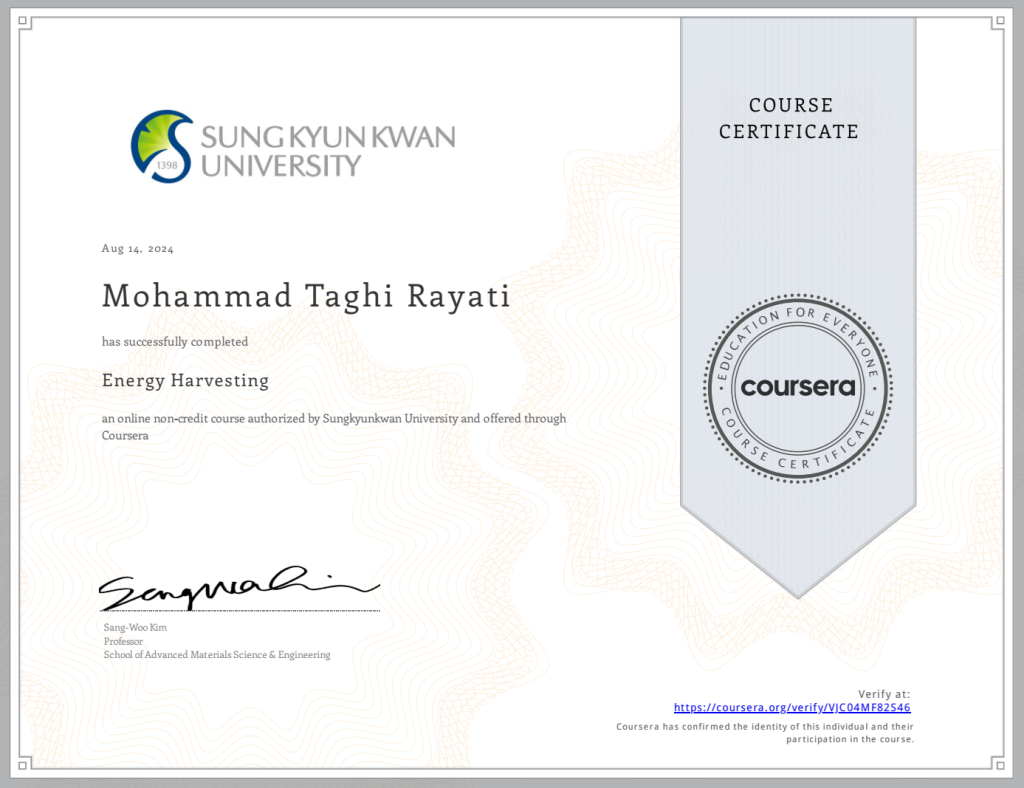
In the “Energy Harvesting” course, I explored various methods of converting and reclaiming lost energy, starting with fundamental concepts like the Carnot cycle, perpetual motion, and the conservation of energy. I examined how entropy and system surroundings play a role in energy transfer and delved into the microscopic aspects of materials such as the band theory and density of states. I also learned about specific phenomena like grain boundary creep fracture, which affects the mechanical properties of materials. The course also highlighted how these principles apply to practical energy harvesting, particularly for Internet of Things (IoT) devices, where methods like flexoelectricity and static electricity are explored. I analyzed the pros and cons of static electricity in energy harvesting, comparing it with traditional methods through Maxwell’s equations.
Additionally, I focused on triboelectric nanogenerators (TENG) and their working mechanisms, including contact-based and rotational methods. I learned about the efficiency of TENG, understanding key factors like surface charge density, work function, and polarity control, as well as the role of electron trap sites and coupling effects. The course also emphasized the importance of impedance matching for optimal energy harvesting. Beyond energy harvesting, I explored potential applications of TENG technology, such as in antibacterial devices and sensors for pressure and acoustics, showcasing its versatility in bio-applications.
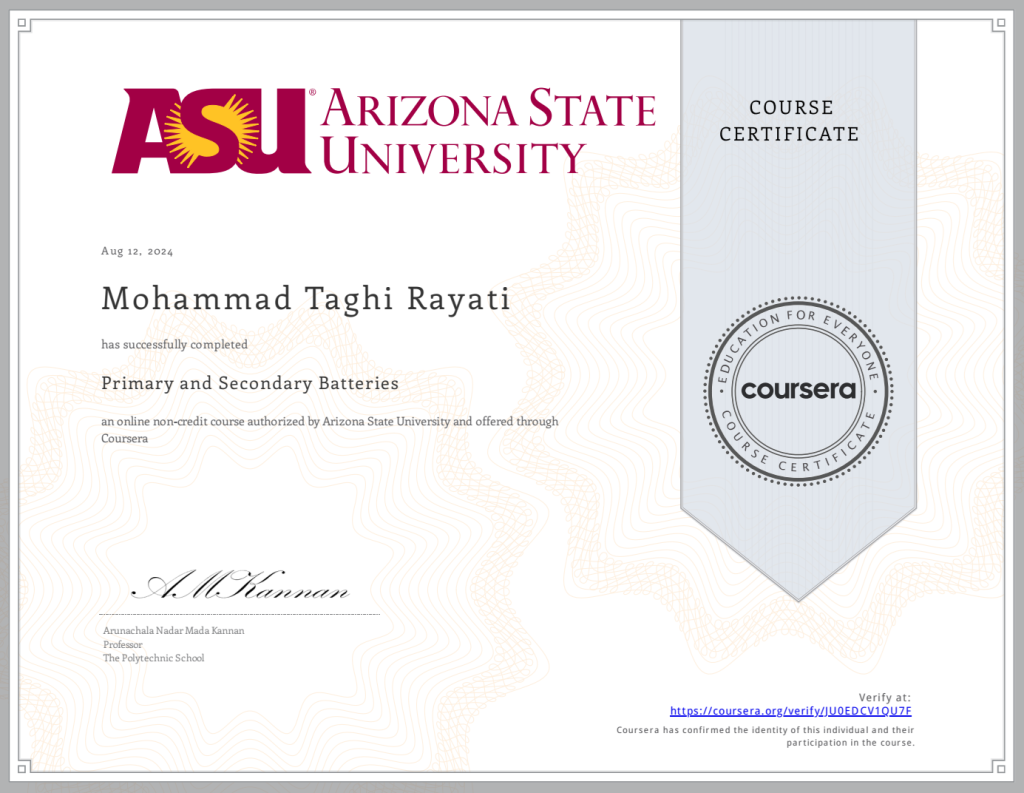
In the “Primary and Secondary Batteries” course, I delved into a comprehensive exploration of battery technology, covering both theoretical and practical aspects. I began with foundational concepts, such as defining battery and cell components and understanding their operating principles. The course offered insights into the differences between primary (single-use) and secondary (rechargeable) batteries, along with essential electrochemical principles like cell potential and the Nernst Equation. I learned how to calculate currents and cell voltages, and grasped the concept of C-Rate, which describes the rate at which a battery is charged or discharged relative to its capacity. Additionally, the course addressed factors affecting battery lifespan, such as environmental conditions, temperature, and depth of discharge.
The course also provided a broad overview of battery applications and the market landscape. I explored the various applications of batteries, from grid-scale energy storage to electric vehicles, and examined market share and pricing trends. Key topics included the goals and standards for battery performance and safety, as well as national and international battery standards. I also learned about battery pack standards and the importance of battery labels and sizes. This well-rounded education equipped me with both theoretical knowledge and practical insights into the evolving field of battery technology.
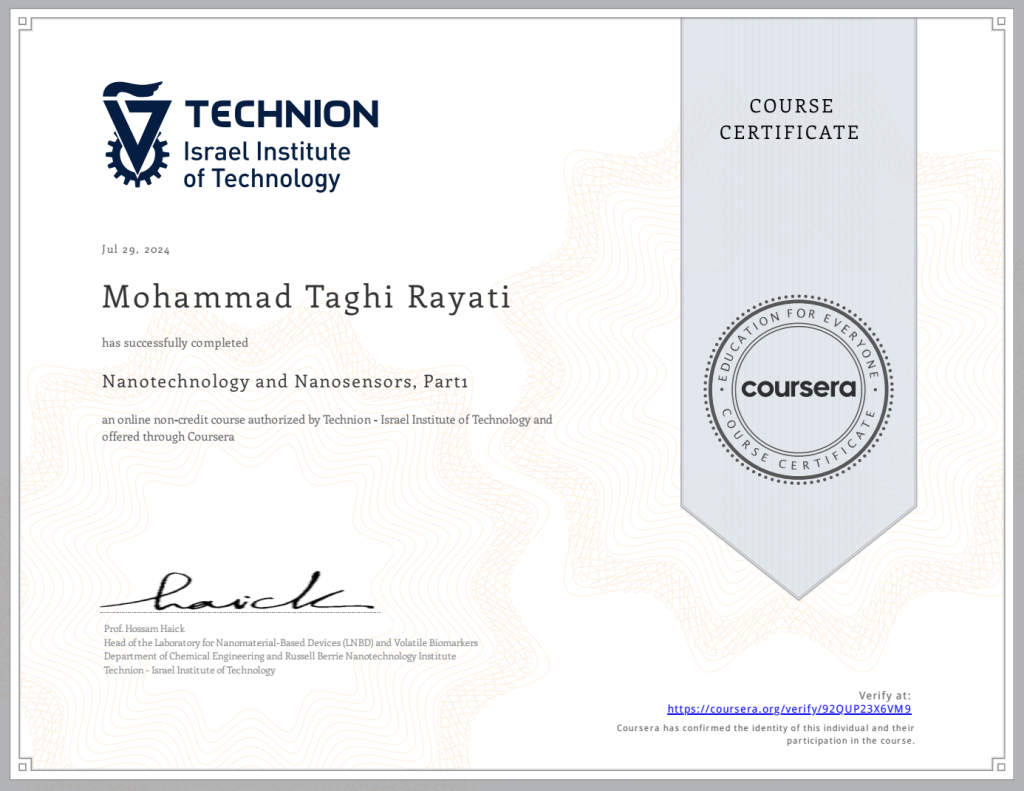
In the “Nanotechnology and Nanosensors, Part 1” course, I gained a comprehensive understanding of nanomaterials and their unique properties, exploring various nanostructures classified by their dimensionality: 0-D, 1-D, 2-D, and 3-D. I learned about the advanced characterization tools essential for analyzing nanomaterials and nanostructures. The course covered fabrication techniques on the nanoscale, including top-down fabrication, which involves etching away material to create nanoscale structures, and bottom-up fabrication, where nanoscale structures are built atom by atom or molecule by molecule. This foundational knowledge was crucial for understanding how nanoscale materials and devices are developed and manipulated.
Furthermore, the course provided an in-depth look at nanosensors, their components, and classification. I studied the parameters essential for sensor characterization and delved into specific types of sensors, such as chemi-resistors based on nanoparticles, and sensors designed for the detection of DNA and biomolecules. I explored the synthesis of nanoparticles and their applications, particularly gold nanoparticles in medical and biological contexts. Additionally, I learned about quantum dots, their properties, synthesis methods, and their use in sensing and imaging applications. This comprehensive overview equipped me with the knowledge to understand and develop advanced nanoscale sensors and their diverse applications in fields ranging from medicine to environmental monitoring.
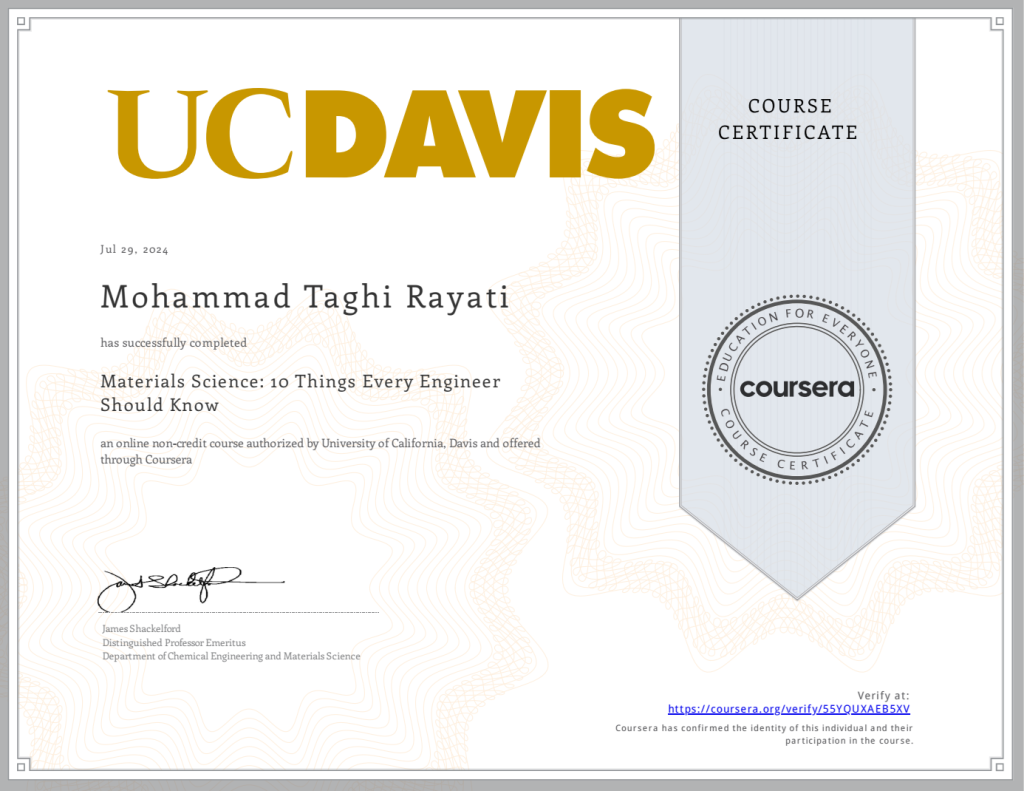
In the course “Materials Science: 10 Things Every Engineer Should Know” I was introduced to the six categories of engineering materials: metals, ceramics, polymers, composites, semiconductors, and biomaterials. Understanding these categories laid the foundation for exploring how the internal structure of materials leads to their properties. I delved into crystallography, learning how the arrangement of atoms within a crystal affects material properties, and utilized electron microscopy to observe these structures. The course also covered the Arrhenius relationship, which explains the temperature dependence of the number of vacancies in a crystal, a crucial factor in understanding material behavior at different temperatures.
Furthermore, I studied various types of defects in materials, such as point defects that facilitate solid-state diffusion and dislocations that explain plastic deformation. The relationship between stress and strain, encompassing the four key mechanical properties—elasticity, plasticity, toughness, and strength—was thoroughly examined. I learned about specific deformation mechanisms like creep, which describes how materials deform under long-term exposure to stress, and the ductile to brittle transition, crucial for predicting material failure. The course also covered fracture toughness, fatigue, phase diagrams, and both diffusional and diffusionless transformations, providing a comprehensive understanding of material behavior. Additionally, semiconductor materials were explored, highlighting their significance in modern electronics. This course equipped me with essential knowledge to analyze and apply materials effectively in engineering contexts.
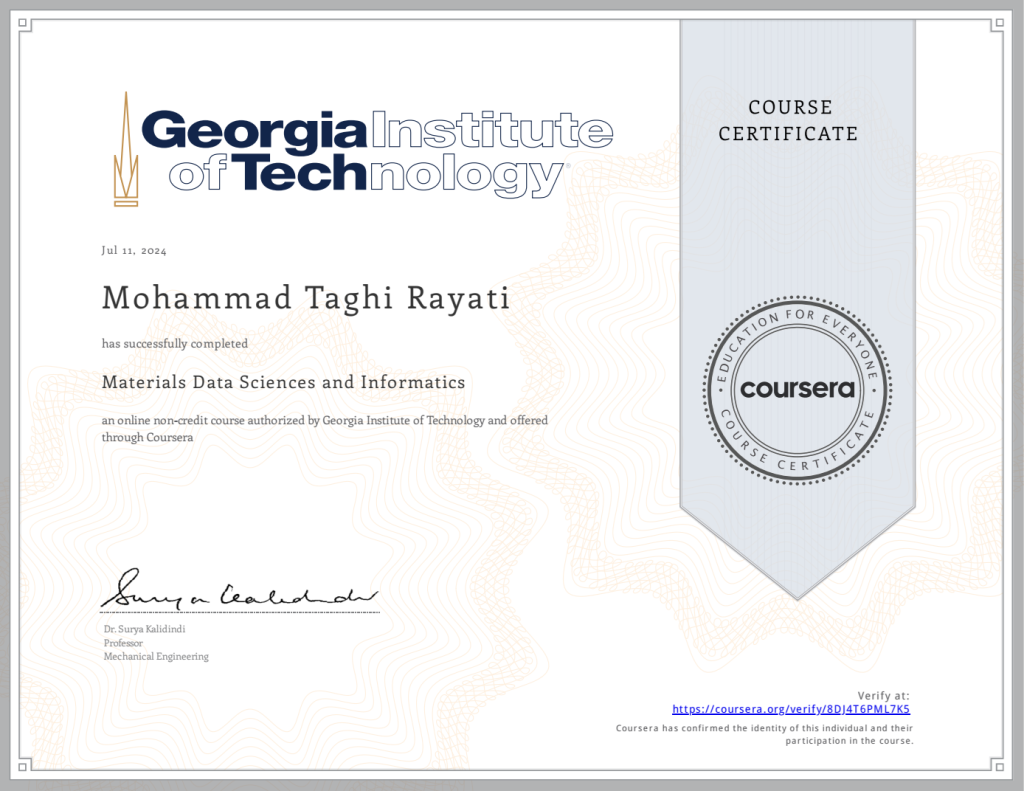
In the “Materials Data Sciences and Informatics” course, I explored several advanced topics essential for modern materials development. I delved into multiscale modeling and multilevel design, learning to predict material behavior across different scales, and studied the Materials Innovation Ecosystem, which emphasizes collaborative efforts to accelerate materials discovery. The Process-Structure-Property (PSP) Linkages framework was a central theme, helping me understand how processing conditions influence microstructure and material properties. Additionally, I learned about the computation and visualization of 2-point spatial correlations to analyze material structures and Principal Component Analysis (PCA) for simplifying complex data to identify key microstructural features.
The course also covered scale-bridging and homogenization techniques to integrate fine-scale information into macroscale models, ensuring accurate material behavior predictions. I gained hands-on experience with PyMKS, an open-source Python library, applying machine learning techniques to study structure-property relationships in materials. This equipped me with the skills to develop data-driven models for material design and innovation, making me proficient in using computational methods and data science tools to address real-world challenges in materials science.
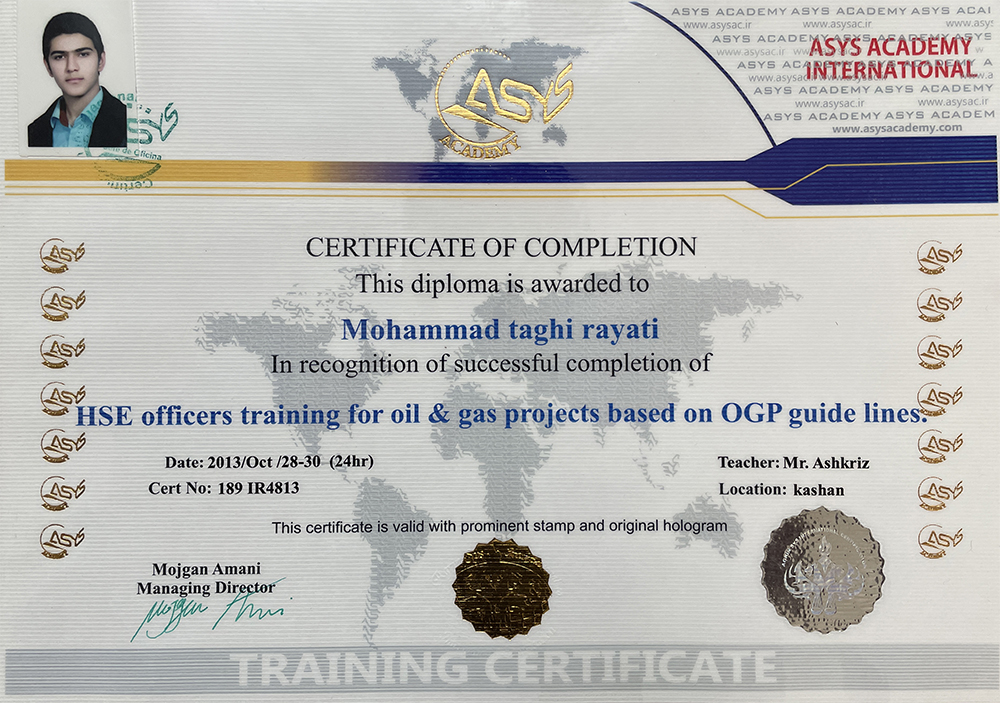
Volunteering Works
- Autism Children Association
collaboration in organizing a charitable event to raise funds for children with autism at the University of Kashan: A pioneering initiative unfolded in Kashan and at the University of Kashan when I, along with a dedicated group of friends, joined forces with the Kashan Autism Association to orchestrate a charitable event within the university’s amphitheater hall. This collective endeavor bore fruit in the form of a newly constructed facility designed to support children with autism. During this event, my central role involved crafting a poignant short film shedding light on the arduous lives of these children and their families. The aim was to create awareness within our society about autism and the challenges it presents.
- City’s Blind Community
Collaborating closely with the City’s Blind Community Association, we worked together on the design and promotion of their Instagram page. Our shared goal was to enhance the visibility of exquisite handicrafts crafted by the visually impaired, ultimately reaching a wider audience and showcasing their incredible talents.